Bensu Sıla Çelik T., Elif DuymazE.
Chimeric antigen receptors (CARs) are synthetic receptors that function to reprogram immune cells, particularly T cells, to recognize and kill cells expressing specific antigens1. CAR-T cells are T cells engineered to combine T cell activation and antigen binding function in a single receptor. This emerging type of therapy was the first cell therapy approved for cancer treatment and the first gene therapy approved by the US Food and Drug Administration (FDA)2,3.
CAR-T cells are engineered to express the synthetic receptors of T cells consisting of three key regulatory elements: The first element is an extracellular single-chain variable fragment that provides target recognition specificity and is derived from the target binding domain of antibodies. The second element contains a hinge domain and transmembrane spacer that provide flexibility and stability. The third element is an intracellular domain that triggers a signaling cascade that promotes T-cell survival, proliferation, and cytotoxicity4. CAR contains a specific antigen binding domain (scFV) that activates T cells independently of the major histocompatibility complex 1 (MHC1). CAR, created by manipulation of T cell receptor (TCR) subunits, is designed for use in cancer therapy and may also be referred to as immunotherapy, gene therapy, or cancer therapy5.
Treatment with CAR-T cells has recently attracted considerable interest around the world. Studies with CAR-T cell therapy include research on many types of cancer; however, recurrence in patients after remission after treatment suggests that the treatment is not completely curative. This recurrence can be explained by tumor antigen escape and inefficient CAR-T cell trafficking to the tumor site6. CAR-T cell therapy has demonstrated its efficacy in hematological malignancies, especially in infants, and cures of up to 81% have been reported in B-cell acute lymphoblastic leukemia (B-ALL)7,8.
For the first time in 2012, CD19-targeted CAR-T cell therapy was tested in follicular lymphoma and chronic lymphocytic leukemia (CLL) types followed in adult patients, and successful results were obtained. Following this success, it was applied to pediatric patients with ALL, and remission was observed after a remarkable improvement. In cases of relapse following treatments, success has been achieved with early phase trials of anti-CD19 CAR-T cells that suppress CD19-expressing B cell malignancies9. In this review, an overview of CAR-T cell engineering, CAR generations, and CAR-T cell-based therapies will be provided.
CAR-T Cell Engineering
The expression of the first synthetic immunoglobulin/TCR chimeric molecule with antibody-like specificity was reported more than 30 years ago10,11. The first involves the interaction between the tissue-compatibility complex expressed on large antigen-presenting cells and the TCR on T cells. This interaction enables physiological or pathological recognition of antigens. In the case of pathological recognition of antigen, the second signal is carried out through co-receptors, cytokines, chemokines, and various molecules12. CARs have an advantage over TCRs because they can recognize antigens without MHC, making them bioengineered receptors with specificity for a targeted antigen13. Gene transfer techniques are used to direct CARs to target specific antigens and ensure their persistent expression on T cells (Figure 1)16. Modulation of CAR-T cell activity regulates the presence of functional CARs on the surface of engineered T cells by adjusting the stability or conformation of the CAR protein itself14. In CAR-T cell-associated cancer studies, promising bioengineering strategies are being developed to reduce toxicities and increase therapeutic efficacy15.
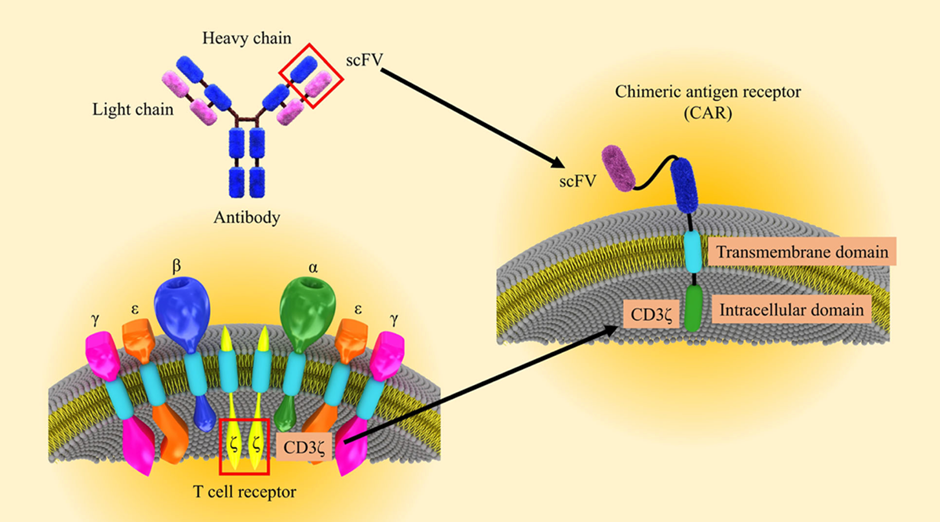
Figure 1. Schematic illustration of CAR-T engineering16. The CAR-T cell is engineered by combining existing immune cell components to facilitate direct targeting of tumor antigens.
CAR Structure and CAR-T Cell Generations
The overall structural organization of CARs consists of an extracellular antibody fragment domain that recognizes antigens, a transmembrane domain, and an intracellular activation signaling domain that activates T cells (Figure 2)17. First-generation CAR-T cells were designed to possess scFv that can bind to extracellular tumor-associated antigens (TAAs) and trigger intracellular signaling from the T cell surface glycoprotein CD3ζ (CD3 zeta) chain domain18. Intracellular signaling of CD3ζ in these first-generation CARs lacked the potency to induce T cell proliferation and long-term anticancer activity, resulting in both CAR-T cell exhaustion and only a modest reduction in tumor growth in vivo19. Efforts to enhance intracellular signaling led to the development of second-generation20 CARs containing a co-stimulatory signal from CD28, followed by third-generation CARs containing multiple co-stimulatory elements, including CD28 as well as 4-1BB or OX40. A more advanced fourth generation of CARs was achieved by adding to co-stimulatory signals the presence of transcription factors such as NFAT (nuclear factor of activated T cells), which can activate the innate immune response through the production and secretion of proinflammatory cytokines21.
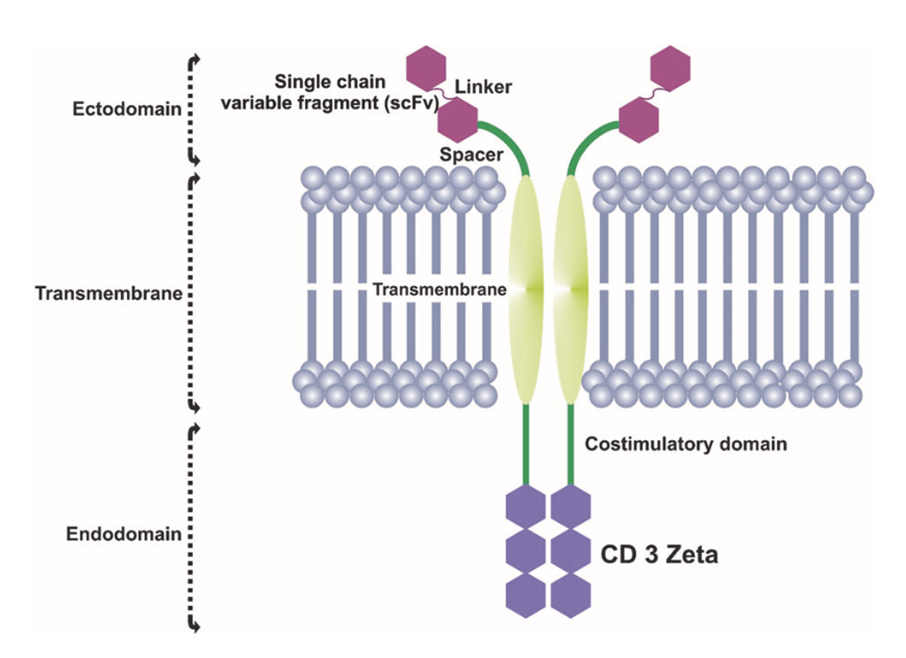
Figure 2. CAR structural organization17. scFV encompasses an extracellular MHC-independent antigen-binding ectodomain derived from a monoclonal antibody, including a linker and a spacer/hinge region, a transmembrane domain, and an intracellular T cell signaling endodomain counting CD3Z and costimulatory domains.
Functional Mechanisms of CAR-T Cells
The choice of target antigen for CAR-T cell therapy is decisive. CARs combine T cell cytotoxicity with antigens of the same origin, independent of the type of cell presenting the antigen22. In other words, CARs, which transform the antigen recognition step required for effective T cell activation into a signaling cascade, combine the cytotoxic effect of the T cell with the specificity of the antigen recognition site23.
Mechanistically, CAR-T cells are different from T cells in that they are created with enhanced anti-tumor properties. It has been reported that CAR-T cells show better results when they work together with helper molecules. For example, it has been discovered that CAR-T cells have a longer duration of action and have a more effective effect on the tumor when working with the STAT5 signaling pathway. In addition to signaling molecules, CAR-T cells can also recognize antigens of structures such as proteins, carbohydrates, and glycolipids24.
Autologous and Allogeneic CAR-T Cell Therapies
The first of the two approaches used for CAR-T cell therapy is autologous (patient-derived) CAR-T cell therapy. This type of therapy is thought to be able to treat relapsed or refractory ALL, relapsed or refractory diffuse large B-cell lymphoma, and primary mediastinal large B-cell lymphoma, types of cancer that have so far been incurable25. Although autologous CAR-T cell therapy sheds light on cancer, it has certain disadvantages. It requires the collection of T cells, the production of a genetically appropriate product for each patient, and full-release testing, which prolongs the process and increases the cost. For these reasons, the success rate of the treatment decreases26.
A second type of treatment, allogenic (donor-derived) CAR-T cell therapy, is much more advantageous than autologous CAR-T cell therapy. The first reason for this advantage is that T cells are more suitable and tolerant for ex vivo manipulation, as they are not exposed to multiple anti-leukemia treatments. The second reason is that there is no time problem as the cells are pre-supplied and prepared in advance. The third reason is that there is no risk of contamination. The last reason is that it is very economically viable27. For these reasons, allogenic CAR-T cell therapy has proven to be more effective and has become a promising treatment method28.
CAR-T cell therapy, which consists of modifying T cells with CARs, is a promising new technique for cancer treatments. Approved in 2017 by the FDA for the first time, the treatment has shown positive results in pediatric and adult cases; however, there are still some ongoing technical limitations. With the emergence of advanced cellular manufacturing techniques, novel cellular engineering approaches, precision genome editing technologies, and combination therapy strategies, CAR-T cells are expected to soon become a readily available, cost-effective, and potentially curative human treatment.
References:
- Sterner, R. C., & Sterner, R. M. (2021). CAR-T cell therapy: current limitations and potential strategies. Blood Cancer Journal 2021 11:4, 11(4), 1–11. https://doi.org/10.1038/s41408-021-00459-7
- Li, R., Ma, C., Cai, H., Chen, W., Li, R., Ma, C., Chen, W., & Cai, H. (2020). The CAR T-Cell Mechanoimmunology at a Glance. Advanced Science, 7(24), 2002628. https://doi.org/10.1002/ADVS.202002628
- Brown, C. E., & Mackall, C. L. (2019). CAR T cell therapy: inroads to response and resistance. Nature Reviews Immunology 2019 19:2, 19(2), 73–74. https://doi.org/10.1038/s41577-018-0119-y
- Abate-Daga, D., & Davila, M. L. (2016). CAR models: Next-generation CAR modifications for enhanced T-cell function. Molecular Therapy – Oncolytics, 3, 16014. https://doi.org/10.1038/mto.2016.14
- Mohanty, R., Chowdhury, C. R., Arega, S., Sen, P., Ganguly, P., & Ganguly, N. (2019). CAR T cell therapy: A new era for cancer treatment (Review). Oncology reports, 42(6), 2183–2195. https://doi.org/10.3892/or.2019.7335
- Poorebrahim, M., Melief, J., Pico de Coaña, Y., L. Wickström, S., Cid-Arregui, A., & Kiessling, R. (2021). Counteracting CAR T cell dysfunction. Oncogene 2020 40:2, 40(2), 421–435. https://doi.org/10.1038/s41388-020-01501-x
- Ma, S., Li, X., Wang, X., Cheng, L., Li, Z., Zhang, C., Ye, Z., & Qian, Q. (2019). Current progress in car-t cell therapy for solid tumors. International Journal of Biological Sciences, 15(12), 2548–2560. https://doi.org/10.7150/IJBS.34213
- Huang, R., Li, X., He, Y., Zhu, W., Gao, L., Liu, Y., Gao, L., Wen, Q., Zhong, J. F., Zhang, C., & Zhang, X. (2020). Recent advances in CAR-T cell engineering. Journal of hematology & oncology, 13(1), 86. https://doi.org/10.1186/s13045-020-00910-5
- Shah, N. N., & Fry, T. J. (2019). Mechanisms of resistance to CAR T cell therapy. Nature Reviews Clinical Oncology 2019 16:6, 16(6), 372–385. https://doi.org/10.1038/s41571-019-0184-6
- Kuwana, Y., Asakura, Y., Utsunomiya, N., Nakanishi, M., Arata, Y., Itoh, S., Nagase, F., & Kurosawa, Y. (1987). Expression of chimeric receptor composed of immunoglobulin-derived V resions and T-cell receptor-derived C regions. Biochemical and Biophysical Research Communications, 149(3), 960–968. https://doi.org/10.1016/0006-291X(87)90502-X
- Gross, G., Waks, T., & Eshhar, Z. (1989). Expression of immunoglobulin-T-cell receptor chimeric molecules as functional receptors with antibody-type specificity. Proceedings of the National Academy of Sciences, 86(24), 10024–10028. https://doi.org/10.1073/PNAS.86.24.10024
- Hwang, J. R., Byeon, Y., Kim, D., & Park, S. G. (2020). Recent insights of T cell receptor-mediated signaling pathways for T cell activation and development. Experimental & Molecular Medicine 2020 52:5, 52(5), 750–761. https://doi.org/10.1038/s12276-020-0435-8
- Tokarew, N., Ogonek, J., Endres, S., von Bergwelt-Baildon, M., & Kobold, S. (2018). Teaching an old dog new tricks: next-generation CAR T cells. British Journal of Cancer 2018 120:1, 120(1), 26–37. https://doi.org/10.1038/s41416-018-0325-1
- Hong, M., Clubb, J. D., & Chen, Y. Y. (2020). Engineering CAR-T Cells for Next-Generation Cancer Therapy. Cancer Cell, 38(4), 473–488. https://doi.org/10.1016/J.CCELL.2020.07.005
- Roselli, E., Faramand, R., & Davila, M. L. (2021). Insight into next-generation CAR therapeutics: designing CAR T cells to improve clinical outcomes. The Journal of Clinical Investigation, 131(2). https://doi.org/10.1172/JCI142030
- Marofi, F., Motavalli, R., Safonov, V. A., Thangavelu, L., Yumashev, A. V., Alexander, M., Shomali, N., Chartrand, M. S., Pathak, Y., Jarahian, M., Izadi, S., Hassanzadeh, A., Shirafkan, N., Tahmasebi, S., & Khiavi, F. M. (2021). CAR T cells in solid tumors: challenges and opportunities. Stem Cell Research & Therapy 2021 12:1, 12(1), 1–16. https://doi.org/10.1186/S13287-020-02128-1
- Sheykhhasan, M., Manoochehri, H., & Dama, P. (2022). Use of CAR T-cell for acute lymphoblastic leukemia (ALL) treatment: a review study. Cancer Gene Therapy 2021 29:8, 29(8), 1080–1096. https://doi.org/10.1038/s41417-021-00418-1
- Zhang, C., Liu, J., Zhong, J. F., & Zhang, X. (2017). Engineering CAR-T cells. Biomarker Research, 5(1). https://doi.org/10.1186/S40364-017-0102-Y
- Subklewe, M., Von Bergwelt-Baildon, M., & Humpe, A. (2019). Chimeric Antigen Receptor T Cells: A Race to Revolutionize Cancer Therapy. Transfusion Medicine and Hemotherapy, 46(1), 15. https://doi.org/10.1159/000496870
- Perica, K., Curran, K. J., Brentjens, R. J., & Giralt, S. A. (2018). Building a CAR Garage: Preparing for the delivery of commercial CAR T products at Memorial Sloan Kettering Cancer Center. Biology of Blood and Marrow Transplantation : Journal of the American Society for Blood and Marrow Transplantation, 24(6), 1135. https://doi.org/10.1016/J.BBMT.2018.02.018
- Tian, Y., Li, Y., Shao, Y., & Zhang, Y. (2020). Gene modification strategies for next-generation CAR T cells against solid cancers. Journal of Hematology & Oncology, 13(1). https://doi.org/10.1186/S13045-020-00890-6
- Hou, A. J., Chen, L. C., & Chen, Y. Y. (2021). Navigating CAR-T cells through the solid-tumour microenvironment. Nature Reviews Drug Discovery 2021 20:7, 20(7), 531–550. https://doi.org/10.1038/s41573-021-00189-2
- Labanieh, L., Majzner, R. G., & Mackall, C. L. (2018). Programming CAR-T cells to kill cancer. Nature Biomedical Engineering 2018 2:6, 2(6), 377–391. https://doi.org/10.1038/s41551-018-0235-9
- Shademan, B., Karamad, V., Nourazarian, A., & Avcı, C. B. (2022). CAR T Cells: Cancer Cell Surface Receptors Are the Target for Cancer Therapy. Advanced Pharmaceutical Bulletin, 12(3), 476. https://doi.org/10.34172/APB.2022.051
- Depil, S., Duchateau, P., Grupp, S. A., Mufti, G., & Poirot, L. (2020). ‘Off-the-shelf’ allogeneic CAR T cells: development and challenges. Nature Reviews Drug Discovery 2020 19:3, 19(3), 185–199. https://doi.org/10.1038/s41573-019-0051-2
- DiNofia, A. M., & Grupp, S. A. (2021). Will allogeneic CAR T cells for CD19+ malignancies take autologous CAR T cells ‘off the shelf’? Nature Reviews Clinical Oncology 2021 18:4, 18(4), 195–196. https://doi.org/10.1038/s41571-021-00485-1
- Qasim, W. (2019). Allogeneic CAR T cell therapies for leukemia. American Journal of Hematology, 94(S1), S50–S54. https://doi.org/10.1002/AJH.25399
- Kim, D. W., & Cho, J. Y. (2020). Recent Advances in Allogeneic CAR-T Cells. Biomolecules 2020, Vol. 10, Page 263, 10(2), 263. https://doi.org/10.3390/BIOM10020263