Gülşah AkçadağA., Elif AltayE.
Pichia pastoris has been described as Zygosaccharomyces pastoris by Alexandre Guilliermond who is French mycologist in 19201. After, similar strains were isolated from oak trees by Herman Phaff and given the name P. pastoris 30-40 years later. Nevertheless, a reclassification occurred in 1995, relocating P. pastoris to a distinct genus identified as Komagataella1,2.
P. pastoris is one of the organisms used as a model organism in molecular biology studies, and there are different important reasons for this. At the beginning, bioproducts which are vaccines, insulin, proteins, and antibodies can be produced on a large scale with low cost since it has high cell density capacity, rapid expression and practical downstream process3,4. Moreover, the genome of P. pastoris is fully sequenced and it is easy to manipulate genetically5. Microbial contamination risk is less with P. pastoris thanks to using methanol as a carbon source. In addition to this information, P. pastoris has post-translational modification which is an essential property in case of heterologous protein production. Therefore, P. pastoris is one of the most predominant yeast species in the case of heterologous protein production in laboratory and industrial scales according to the results of literature studies6.
Pichia pastoris Genetic Background
P. pastoris strains are grouped into two different species as Komagataella pastoris and K. phaffii. However, P. pastoris name is preferred for both species since various strains are under the Komagataella genus1. P. pastoris is a methylotrophic yeast which means it can utilize methanol as a carbon and energy source6. In addition, methanol utilization capacity based on alcohol oxidase genes (AOX1 and AOX2) of P. pastoris determines phenotype7. If P. pastoris has both AOX1 and AOX2 genes, then it is called wild type strains (Mut+) and it can grow on methanol. Mut-strains cannot utilize methanol since it does not contain AOX1 and AOX2 genes. Additionally, there is a MutS phenotype that can grow on methanol slowly since one or more alcohol oxidase genes are deleted6. K. pastoris which is a Mut+ and its ATCC number is 28485 has 4 chromosomes7,8. In addition to this information, its genome size is 9.6 Mb, and it can code 5241 genes with 41.5% GC content9,10.
Different strains of P. pastoris and their features are summarized in Table 1.
Strain | Genotype | Phenotype | Protease Deficiency |
X-33 | WT | Mut+ | – |
GS115 | his4 | Mut+, His- | – |
KM71 | ∆aox1::SARG4 his4 arg4 | Muts, His- | + |
SMD1165 | His4 prb1 | Mut+His–Prot–(B–) | + |
SMD1168 | his4, ura3, pep4::URA3 | (A–, BS, CarbY–) | + |
Pichia pastoris as An Expression System
P. pastoris has various advantages as an expression system. There are strong promoters as Glyceraldehydes-3-phosphate dehydrogenase (GAP), formaldehyde dehydrogenase (FLD), and peroxisomal matrix protein (PEX8) to use in the P. pastoris expression system6,11,12. Most importantly, it can express genes under the AOX1 promoter which is one of the strongest and closely regulated eukaryotic promoters according to literature studies13. Methanol can be used as an inducer in transcription owing to the AOX1 promoter. Various protein yields reach 14.8 g/L under the expression AOX1 promoter14. Moreover, dry weight reaches 200 g/L in the case of acetic acid and ethanol production15.
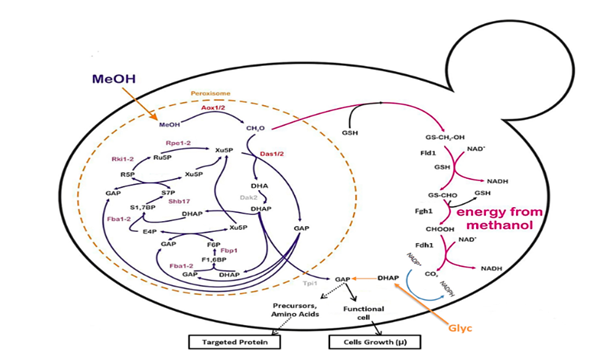
Figure 2. Methanol utilization pathway of P. pastoris16.
Figure 2 illustrates the crucial roles of AOX1 and AOX2 in the oxidation of methanol to formaldehyde. Subsequently, formaldehyde becomes a key player in either the assimilatory or dissimilatory pathways. When formaldehyde participates in assimilatory pathways, it leads to the formation of glyceraldehyde-3-phosphate (GAP) and dihydroxyacetone (DHA), contributing to biomass generation through various reactions. On the other hand, if formaldehyde is directed towards the dissimilation pathway, each mole of methanol yields 2 moles of nicotinamide adenine dinucleotide hydrogen (NADH), accompanied by the release of CO2 through formaldehyde oxidation16,17.
Moreover, AOX1 promoter can be repressed through various carbon sources such as glycerol and glucose17. Therefore, high biomass can be obtained since the initial carbon source prevents transcription of the gene. Instead of these advantages, AOX1 promoter usage can be harmful on an industrial scale, since methanol can cause toxic effects and fire6. Remarkably, AOX1 promoter is one of the strongest promoters in the case of heterologous gene expression in literature18.
There are synthetic and drug-resistance markers in the P. pastoris expression system. As an example, HIS4, ARG4 and URA3 are synthetic markers while kanamycin and zeocin are drug-resistances19. However, these markers have their own features, and the production process must be planned according to them.
P. pastoris secretion capacity is an essential point in the case of heterologous protein production. P. pastoris has Saccharomyces cerevisiae α-mating factor prepro-peptide, and it is the most frequently used secretion signal leader in the case of P. pastoris20. Indeed, purification steps will be easier and cheaper with P. pastoris expression system thanks to having secretion signal peptide and not having many native secreted proteins6.
Plasmid durability is another important point in the case of expression systems. Plasmids can lose cell division in time. Therefore, it is a bottleneck in the case of heterologous protein productions. P. pastoris expression system has an essential feature, which is called genome integration. Genome integration is an outstanding property owing to sustainability, and it can be achieved through three main protocols electroporating, spheroplast formation, and lithium chloride treatment21. There are different regions for this purpose on P. pastoris chromosome as HIS4 and AOX1 locus. However, AOX1 locus is one of the most chosen loci in the case of homologous recombination21,22. For this purpose, AOX1 locus is preferred frequently since its expression level is high and can be tightly regulated through methanol induction23. In addition, this phenomenon is used as an expression cassette and provides various heterologous protein production.
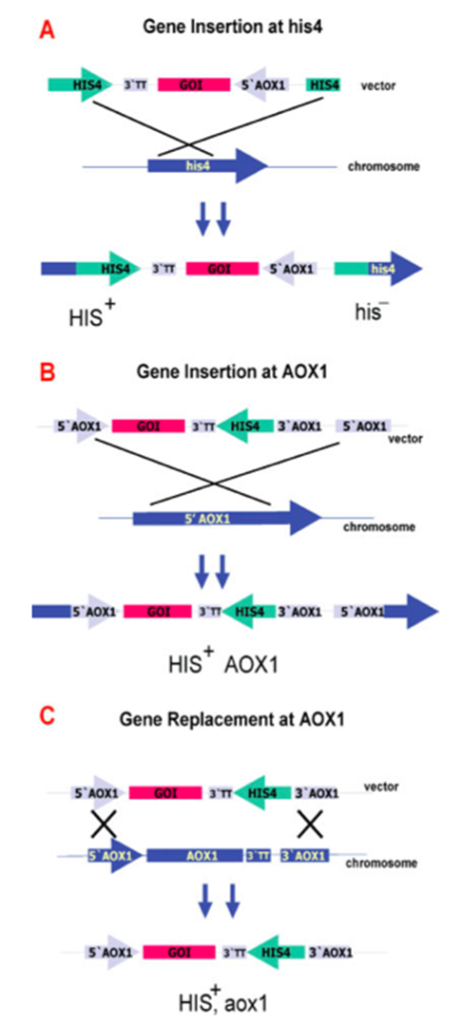
Figure 3. Genome Integration in P. pastoris21. A. Gene insertion at HIS4 B. Gene insertion at AOX1 C. Gene replacement at AOX1.
P. pastoris has valuable advantages over other expression systems such as bacterial or mammalian. Post-translational modifications, which are most importantly glycosylation, phosphorylation, and acetylation are important in the case of heterologous protein production23.
These modifications can be achieved through mammalian expression systems while it cannot fully perform in the case of bacterial expression systems. Therefore, misfolded, and insoluble proteins are produced in bacterial expression systems21,24. P. pastoris expression system provides post-translational modifications. Indeed, desired protein production is achieved with correct folding20. In contrast to the bacterial expression system, secretion signal capacity is a precisive property for P. pastoris system. In mammalian expression systems, post-translational modifications can be done correctly but the system is more time consuming than yeast expression systems owing to long doubling time. Moreover, mammalian expression systems are more expensive than bacterial and yeast systems because of complex growth medium23.
Usage Areas of Pichia pastoris
P. pastoris is frequently used in laboratory and industrial scales owing to its high concentration yield. There are enormous biomolecules that are produced through this expression system24.
Industrial Enzymes
According to literature studies’ results, biomolecules that are produced P. pastoris expression system are used in different industries.
As an example, wastewater treatment is an essential topic nowadays. Ligninolytic enzymes are mostly preferred in the case of wastewater treatment. Since P. pastoris does not produce cellulolytic enzymes in contrast to other filamentous fungi, produced ligninolytic enzymes can be applied to related industries without extra purification steps. Additionally, different sources of laccase genes that encode ligninolytic enzymes are successfully produced with high activity yield in P. pastoris expression system24-26.
Moreover, there are a lot of essential enzymes that are used in different industry areas such as paper, textile, detergent, food and beverage, wine, and pharmaceuticals. Cellulases, xylanases, proteases, esterases, lipases, amylases, phosphatases, and pectinase are the most frequently used industrial enzymes 25,26. Most of these enzymes are produced through the P. pastoris expression system as shown in Table 2. According to Table 2, fed-batch fermentation is preferred to produce these enzymes, and yield ranges vary due to activity calculations27-34.
Table 2. Bioproducts which are Produced Through P. pastoris Expression System27-34.
Product Name | Pichia species/strain | Fermentation Type | Titer/Yield | Reference |
Laccase | P. pastoris | Fed-batch | 0.495 g/L | 27 |
Lipase | P. pastoris | Fed-batch | 2 g/L | 28 |
Phytase | P. pastoris | Fed-batch | 5 g/L | 29 |
Cellulase | P. pastoris | Fed-batch | 2.75 g/L | 30 |
Transglutaminase | GS115 | Batch | 0.70 U/mL | 31 |
Pectate lyase | P. pastoris | Fed-batch | 9.5 g/L | 32 |
α-Amylase | X-33 | Fed-batch | 750 U/mL | 33 |
Trypsin | GS115 | Fed-batch | 177.85 U/mL | 34 |
Pectinase occurs in three main pectinolytic enzymes as polygalacturonase, pectin methyl esterase, and pectin lyase, and these enzymes have a role in the degradation of pectin according to their reactions25,35. Pectinolytic enzymes are used in different industries such as fruit juice since they can decrease viscosity, remove gel structure, and increase the stability of fruit juice36. Various results are related to producing pectinases through the P. pastoris expression system. For instance, high-level expression of Talaromyces celluolyticus endo-polygalacturonase is achieved through P. pastoris in 201937. In the study, five copies of the gene of interest are integrated into P. pastoris genome, and enzyme activity is reached 7124.8 U/mL with fed-batch cultivation37.
Biofuels
Biofuels are a hot topic worldwide due to the limited energy sources and environmental pollution. In this correction, alternative energy sources studies are increasing day by day. Various biofuels which are biodiesel and bioethanol are produced through the P. pastoris expression system. According to the literature, separate hydrolysis and fermentation techniques are used to get bioethanol (14.36 g/L) with P. stipites30,38. In addition to these, there is another study that is related to bioethanol production (37 g/L) through P. fermentas batch fermentation30,39. Moreover, there is a study which is related to the co-culture method. In this study, recombinant strains of P. pastoris and Saccharomyces cerevisiae are used to produce bioethanol. In this study, 32 g/L bioethanol production is achieved, and this concentration corresponds to %82 of the theoretical yield according to study results40.
Biopharmaceuticals
P. pastoris is frequently used in the biopharmaceutical field to produce diverse products, including vaccines, hormones, cytokines, and antibodies3,4. Notably, insulin, a pivotal hormone with significant implications for various medical conditions, is synthesized using distinct strains of P. pastoris, such as X33, GS115, and SuperMan541,42,43. Immunoglobulin G, a crucial antibody, can be generated with yields of up to 227 mg/L through the utilization of diverse strains of P. pastoris44,45. Vaccines have remained a perennial subject of interest within the pharmaceutical domain for centuries. In this correction, P. pastoris is used to produce various vaccines such as HBsAg, DENV-3 E and HCV E1E246-49. Cytokines are small proteins that have a role in cell signaling. Their recombinant production is an outstanding topic for the pharmaceutical area. According to literature studies results, interleukin-6 and interleukin-3 are successfully produced through wild-type P. pastoris strains with 280 mg/L and 26 mg/L yields50,51. Moreover, another cytokine, recombinant bovine interferon α1 is produced with GS115 strain of Pichia52.
In conclusion, P. pastoris holds significance as a crucial methylotrophic yeast due to its extensive applications in large-scale industrial biotechnology. It stands out as one of the preferred expression systems owing to its capabilities in high cell density cultivation, cost-effective downstream processes, presence of genomic sequence, genetic manipulability, and facilitation of post-translational modifications.
References:
1. Peña, D. A., Gasser, B., Zanghellini, J., Steiger, M. G., & Mattanovich, D. (2018). Metabolic engineering of Pichia pastoris. Metabolic engineering, 50, 2-15. https://doi.org/10.1016/j.ymben.2018.04.017
2. Joseph, J. A., Akkermans, S., Nimmegeers, P., & Van Impe, J. F. (2019). Bioproduction of the recombinant sweet protein thaumatin: Current state of the art and perspectives. Frontiers in microbiology, 10, 695. https://doi.org/10.3389/fmicb.2019.00695
3. Dai, M., Yu, C., Fang, T., Fu, L., Wang, J., Zhang, J., Ren, J., Xu, J., Zhang, X., & Chen, W. Identification and Functional Characterization of Glycosylation of Recombinant Human Platelet-Derived Growth Factor-BB in Pichia pastoris. PloS one,2015; 10(12), e0145419. https://doi.org/10.1371/journal.pone.0145419
4. Carnicer, M., Canelas, A. B., Ten Pierick, A., Zeng, Z., van Dam, J., Albiol, J., … & van Gulik, W. (2012). Development of quantitative metabolomics for Pichia pastoris. Metabolomics, 8, 284-298. https://doi.org/10.1007/s11306-011-0308-1
5. Küberl, A., Schneider, J., Thallinger, G. G., Anderl, I., Wibberg, D., Hajek, T., … & Pichler, H. (2011). High-quality genome sequence of Pichia pastoris CBS7435. Journal of biotechnology, 154(4), 312-320. https://doi.org/10.1016/j.jbiotec.2011.04.014
6. Kuruti, K., Vittaladevaram, V., Urity, S. V., Palaniappan, P., & Bhaskar, R. U. (2020). Evolution of Pichia pastoris as a model organism for vaccines production in healthcare industry. Gene Reports, 21, 100937. https://doi.org/10.1016/j.genrep.2020.100937
7. Ohi, H., Okazaki, N., Uno, S., Miura, M., & Hiramatsu, R. (1998). Chromosomal DNA patterns and gene stability of Pichia pastoris. Yeast, 14(10), 895-903. https://doi.org/10.1002/(SICI)1097-0061(199807)14:10<895::AID-YEA288>3.0.CO;2-9
8. Sturmberger, L., Chappell, T., Geier, M., Krainer, F., Day, K. J., Vide, U., … & Glieder, A. (2016). Refined Pichia pastoris reference genome sequence. Journal of Biotechnology, 235, 121-131. https://doi.org/10.1016/j.jbiotec.2016.04.023
9. Cereghino, J. L., & Cregg, J. M. (2000). Heterologous protein expression in the methylotrophic yeast Pichia pastoris. FEMS microbiology reviews, 24(1), 45-66. https://doi.org/10.1111/j.1574-6976.2000.tb00532.x
10. Macauley‐Patrick, S., Fazenda, M. L., McNeil, B., & Harvey, L. M. (2005). Heterologous protein production using the Pichia pastoris expression system. Yeast, 22(4), 249-270. https://doi.org/10.1002/yea.1208
11. Li, P., Anumanthan, A., Gao, X. G., Ilangovan, K., Suzara, V. V., Düzgüneş, N., & Renugopalakrishnan, V. (2007). Expression of recombinant proteins in Pichia pastoris. Applied biochemistry and biotechnology, 142, 105-124. https://doi.org/10.1007/s12010-007-0003-x
12. Waterham, H. R., Digan, M. E., Koutz, P. J., Lair, S. V., & Cregg, J. M. (1997). Isolation of the Pichia pastoris glyceraldehyde-3-phosphate dehydrogenase gene and regulation and use of its promoter. Gene, 186(1), 37-44. https://doi.org/10.1016/S0378-1119(96)00675-0
13. Çelik, E., & Çalık, P. (2012). Production of recombinant proteins by yeast cells. Biotechnology advances, 30(5), 1108-1118. https://doi.org/10.1016/j.biotechadv.2011.09.011
14. Werten, M. W., van den Bosch, T. J., Wind, R. D., Mooibroek, H., & de Wolf, F. A. (1999). High‐yield secretion of recombinant gelatins by Pichia pastoris. Yeast, 15(11), 1087-1096. https://doi.org/10.1002/(SICI)1097-0061(199908)15:11<1087::AID-YEA436>3.0.CO;2-F
15. Heyland J, Fu JA, Blank LM, Schmid A. Quantitative physiology of Pichia pastorisduring glucose-limited high-cell density fed-batch cultivation for recombinant protein production. Biotechnol Bioeng 2010;107:357–68. https://doi.org/10.1002/bit.22836
16. Boojari, M. A., Rajabi Ghaledari, F., Motamedian, E., Soleimani, M., & Shojaosadati, S. A. (2023). Developing a metabolic model‐based fed‐batch feeding strategy for Pichia pastoris fermentation through fine‐tuning of the methanol utilization pathway. Microbial Biotechnology. https://doi.org/10.1111/1751-7915.14264
17. Gassler, T., Sauer, M., Gasser, B., Egermeier, M., Troyer, C., Causon, T. et al. (2020) The industrial yeast Pichia pastoris is converted from a heterotroph into an autotroph capable of growth on CO2. Nature Biotechnology, 38, 210 –216. https://doi.org/10.1038/s41587-019-036
18. Cámara, E., Landes, N., Albiol, J., Gasser, B., Mattanovich, D., & Ferrer, P. (2017). Increased dosage of AOX1 promoter-regulated expression cassettes leads to transcription attenuation of the methanol metabolism in Pichia pastoris. Scientific reports, 7(1), 44302. http://doi.org/10.1038/srep44302
19. Weidner, M., Taupp, M., & Hallam, S. J. (2010). Expression of recombinant proteins in the methylotrophic yeast Pichia pastoris. JoVE (Journal of Visualized Experiments), (36), e1862. https://doi.org/10.3791/1862
20. Chahal, S., Wei, P., Moua, P., Park, S. P. J., Kwon, J., Patel, A., … & Lin-Cereghino, G. P. (2017). Structural characterization of the α-mating factor prepro-peptide for secretion of recombinant proteins in Pichia pastoris. Gene, 598, 50-62. https://doi.org/10.1016/j.gene.2016.10.040
21. Daly, R., & Hearn, M. T. (2005). Expression of heterologous proteins in Pichia pastoris: a useful experimental tool in protein engineering and production. Journal of Molecular Recognition: An Interdisciplinary Journal, 18(2), 119-138. https://doi.org/10.1002/jmr.687
22. Schwarzhans, J. P., Wibberg, D., Winkler, A., Luttermann, T., Kalinowski, J., & Friehs, K. (2016). Non-canonical integration events in Pichia pastoris encountered during standard transformation analysed with genome sequencing. Scientific reports, 6(1), 38952. https://doi.org/10.3389/fbioe.2020.00850
23. Karbalaei, M., Rezaee, S. A., & Farsiani, H. (2020). Pichia pastoris: A highly successful expression system for optimal synthesis of heterologous proteins. Journal of cellular physiology, 235(9), 5867-5881.https://doi.org/10.1002/jcp.29583
24. Shekhar, C. (2008). Pichia power: India’s biotech industry puts unconventional yeast to work. Chemistry & biology, 15(3), 201-202. https://doi.org/10.1016/j.chembiol.2008.03.002
25. Rabert, C., Weinacker, D., Pessoa Jr, A., & Farías, J. G. (2013). Recombinants proteins for industrial uses: utilization of Pichia pastoris expression system. Brazilian Journal of Microbiology, 44, 351-356. https://doi.org/10.1590/S1517-83822013005000041
26. Shrivastava, A., Pal, M., & Sharma, R. K. (2023). Pichia as yeast cell factory for production of industrially important bio-products: Current trends, challenges, and future prospects. Journal of Bioresources and Bioproducts. https://doi.org/10.1016/j.jobab.2023.01.007
27. Kittl R, Gonaus C, Pillei C et al (2012) Constitutive expression of Botrytis aclada laccase in Pichia pastoris. Bioengineered. https://doi.org/10.4161/bioe.20037
28. Jahic M, Wallberg F, Bollok M et al (2003) Temperature limited fed-batch technique for control of proteolysis in Pichia pastoris bioreactor cultures. Microb Cell Fact 2:1–11. https://doi.org/10.1186/1475-2859-2-6
29. Chen CC, Wu PH, Huang CT, Cheng KJ (2004) A Pichia pastoris fermentation strategy for enhancing the heterologous expression of an Escherichia coli phytase. Enzyme Microb Technol. https://doi.org/10.1016/j.enzmictec.2004.05.007
30. Thongekkaew J, Ikeda H, Masaki K, Iefuji H (2008) An acidic and thermostable carboxymethyl cellulase from the yeast Cryptococcus sp. S-2: purifcation, characterization and improvement of its recombinant enzyme production by high cell-density fermentation of Pichia pastoris. Protein Expr Purif. https://doi.org/10.1016/j.pep.2008.03.021
31. Yang, X., & Zhang, Y. (2019). Expression of recombinant transglutaminase gene in Pichia pastoris and its uses in restructured meat products. Food Chemistry, 291, 245-252. https://doi.org/10.1016/j.foodchem.2019.04.015
32. Zheng X, Zhang Y, Liu X et al (2020) High-level expression and biochemical properties of a thermo-alkaline pectate lyase from Bacillus sp. RN1 in Pichia pastoris with potential in Ramie degumming. Front Bioeng Biotechnol. https://doi.org/10.3389/fbioe.2020.00850
33. Parashar, D., & Satyanarayana, T. (2017). Production of chimeric acidic α-amylase by the recombinant Pichia pastoris and its applications. Frontiers in microbiology, 8, 493. https://doi.org/10.3389/fmicb.2017.00493
34. Zhang, Y., Huang, H., Yao, X., Du, G., Chen, J., & Kang, Z. (2018). High-yield secretory production of stable, active trypsin through engineering of the N-terminal peptide and self-degradation sites in Pichia pastoris. Bioresource technology, 247, 81-87. https://doi.org/10.1016/j.biortech.2017.08.006
35. Pedrolli, D. B., Monteiro, A. C., Gomes, E., & Carmona, E. C. (2009). Pectin and pectinases: production, characterization and industrial application of microbial pectinolytic enzymes. Open Biotechnology Journal, 9-18. https://doi.org/10.2174/1874070700903010009
36. Garg, G., Singh, A., Kaur, A., Singh, R., Kaur, J., & Mahajan, R. (2016). Microbial pectinases: an ecofriendly tool of nature for industries. 3 Biotech, 6, 1-13. https://doi.org/10.1007/s13205-016-0371-4
37. Peng, X. B., Chen, G. J., Han, Z. G., & Yang, J. K. (2019). High-level secretive expression of a novel achieved Talaromyces cellulolyticus endo-polygalacturonase in Pichia pastoris by improving gene dosage for hydrolysis of natural pectin. World Journal of Microbiology and Biotechnology, 35, 1-12. https://doi.org/10.1007/s11274-019-2657-2
38. Kut, A., Demiray, E., Ertuğrul Karatay, S., & Dönmez, G. (2022). Second generation bioethanol production from hemicellulolytic hydrolyzate of apple pomace by Pichia stipitis. Energy Sources, Part A: Recovery, Utilization, and Environmental Effects, 44(2), 5574-5585. https://doi.org/10.1080/15567036.2020.1838000
39. Shrivastava, A., & Sharma, R. K. (2022). Evaluation of co-culture system to produce ethanol and electricity from wheat straw hydrolysate using Saccharomyces cerevisiae and Pichia fermentans. Biomass Conversion and Biorefinery, 1-10. https://doi.org/10.1007/s13399-022-02914-2
40. Zhang, Y., Wang, C., Wang, L., Yang, R., Hou, P., & Liu, J. (2017). Direct bioethanol production from wheat straw using xylose/glucose co-fermentation by co-culture of two recombinant yeasts. Journal of Industrial Microbiology and Biotechnology, 44(3), 453-464. https://doi.org/10.1007/s10295-016-1893-9
41. Baeshen, M. N., Bouback, T. A., Alzubaidi, M. A., Bora, R. S., Alotaibi, M. A., Alabbas, O. T., … & Baeshen, N. A. (2016). Expression and purification of C-peptide containing insulin using Pichia pastoris expression system. BioMed research international, 2016. https://doi.org/10.1155/2016/3423685
42. Polez, S., Origi, D., Zahariev, S., Guarnaccia, C., Tisminetzky, S. G., Skoko, N., & Baralle, M. (2016). A simplified and efficient process for insulin production in Pichia pastoris. PloS one, 11(12), e0167207. https://doi.org/10.1371/journal.pone.0167207
43. Gurramkonda, C., Polez, S., Skoko, N., Adnan, A., Gäbel, T., Chugh, D., … & Rinas, U. (2010). Application of simple fed-batch technique to high-level secretory production of insulin precursor using Pichia pastoris with subsequent purification and conversion to human insulin. Microbial cell factories, 9(1), 1-11. https://doi.org/10.1186/1475-2859-9-31
44. Wang, D. D., Su, M. M., Sun, Y., Huang, S. L., Wang, J., & Yan, W. Q. (2012). Expression, purification and characterization of a human single-chain Fv antibody fragment fused with the Fc of an IgG1 targeting a rabies antigen in Pichia pastoris. Protein expression and purification, 86(1), 75-81. https://doi.org/10.1016/j.pep.2012.08.015
45. Chen, M. T., Lin, S., Shandil, I., Andrews, D., Stadheim, T. A., & Choi, B. K. (2012). Generation of diploid Pichia pastoris strains by mating and their application for recombinant protein production. Microbial cell factories, 11, 1-18. https://doi.org/10.1186/1475-2859-11-91
46. Gurramkonda, C., Zahid, M., Nemani, S. K., Adnan, A., Gudi, S. K., Khanna, N., … & Rinas, U. (2013). Purification of hepatitis B surface antigen virus-like particles from recombinant Pichia pastoris and in vivo analysis of their immunogenic properties. Journal of Chromatography B, 940, 104-111. https://doi.org/10.1016/j.jchromb.2013.09.030
47. Gurramkonda, C., Adnan, A., Gäbel, T., Lünsdorf, H., Ross, A., Nemani, S. K., … & Rinas, U. (2009). Simple high-cell density fed-batch technique for high-level recombinant protein production with Pichia pastoris: Application to intracellular production of Hepatitis B surface antigen. Microbial Cell Factories, 8, 1-8. https://doi.org/10.1186/1475-2859-8-13
48. Tripathi, L., Mani, S., Raut, R., Poddar, A., Tyagi, P., Arora, U., … & Khanna, N. (2015). Pichia pastoris-expressed dengue 3 envelope-based virus-like particles elicit predominantly domain III-focused high titer neutralizing antibodies. Frontiers in microbiology, 6, 1005. https://doi.org/10.3389/fmicb.2015.01005
49. Cai, W., Su, L., Liao, Q., Ye, L., Wu, Y., Wu, Z., & She, Y. (2010). Expression, purification and immunogenic characterization of hepatitis C virus recombinant E1E2 protein expressed by Pichia pastoris yeast. Antiviral research, 88(1), 80-85. https://doi.org/10.1016/j.antiviral.2010.07.007
50. Li, H., Wang, Y., Xu, A., Li, S., Jin, S., & Wu, D. (2011). Large-scale production, purification and bioactivity assay of recombinant human interleukin-6 in the methylotrophic yeast Pichia pastoris. FEMS yeast research, 11(2), 160-167. https://doi.org/10.1111/j.1567-1364.2010.00701.x
51. Li, H., Li, N., Gao, X., Kong, X., Li, S., Xu, A., … & Wu, D. (2011). High level expression of active recombinant human interleukin-3 in Pichia pastoris. Protein expression and purification, 80(2), 185-193. https://doi.org/10.1016/j.pep.2011.08.027
52. Shao, J., Cao, C., Bao, J., Liu, H., Peng, T., Gao, M., & Wang, J. (2015). Characterization of bovine interferon α1: expression in yeast Pichia pastoris, biological activities, and physicochemical characteristics. Journal of Interferon & Cytokine Research, 35(3), 168-175. https://doi.org/10.1089/jir.2013.0139