Esma TopaloğluT., Gülnur UzunE.
A multidisciplinary approach has become the main feature of scientific research that points to benefit from the diversity of information of complex systems on or outside of Earth, like biodiversity, ecological systems, and galaxies1. One of the biggest problems in the analysis and interpretation of ecological data is to enlighten the time-space relationship of biosystems2. Difficulty in ecosystem studies defined as “Vectors arranged in multi-dimensional plane with heterogenous surface” is frequently faced by the astronomy field and this has led to the formation of a scientific collaboration called Astroecology1.
Ecology is derived from Greek words “oikos” (home) and “logos” (information)3, and is a science branch that inspects organism variety, organisms, and their relationship with their abiotic and biotic environment4,5, whereas astroecology is a science branch that studies the relationship of life and cosmic environment between potential biotas (whole organisms in a specific geographic area) and resources found in space6. For a deep understanding of ecology, forms of living should be identified and the amount of substances should be quantified or estimated. To determine the lifetime of ecologic studies, we should first answer the question “What is life?”7. Even though this question is not properly answered yet, there are different approaches in terms of exergy (present energy of ecosystem components), entropy (metric of abundance and distribution), and information, that explain the cycle and balance of life in ecosystems8-10. Origin of organic life research is based on the RNA (ribonucleic acid) world hypothesis and focuses on the expansion of biological, genetic, and chemical life of living things. Abiotic nucleotide synthesis which came at the beginning of these processes, is a difficult research topic in prebiotic chemistry11. Panspermia theory expresses the possibility that life came to our planet by comets and asteroids12,13. This theory has pushed scientists to search other planets14,15 with signs of life, and later, microbial ecology16, theoretical ecology models17, and forming ecologic scenarios18 for the transfer of life between planets. In this review, the protection of biodiversity on Earth and experimental and potential astroecology approaches for extraterrestrial colonization will be discussed.
Space and Ecology Relationship
The environment of space is characterized by various stress factors for living such as intense radiation, microgravity, heat, and pressure effects. Effects of stress factors, alone or in combination, may result in modification of genetics of living and evolutionary differences that would not be possible in Earth’s gravity19. For example, an astroecology experiment made by Mautner and his colleagues demonstrated that asparagus (Asparagus sp.) cultures treated with nutrients obtained from carbonaceous chondrites (meteorite piece) and Mars meteorites, increased plant growth because of the high phosphate content (Figure 1)7.
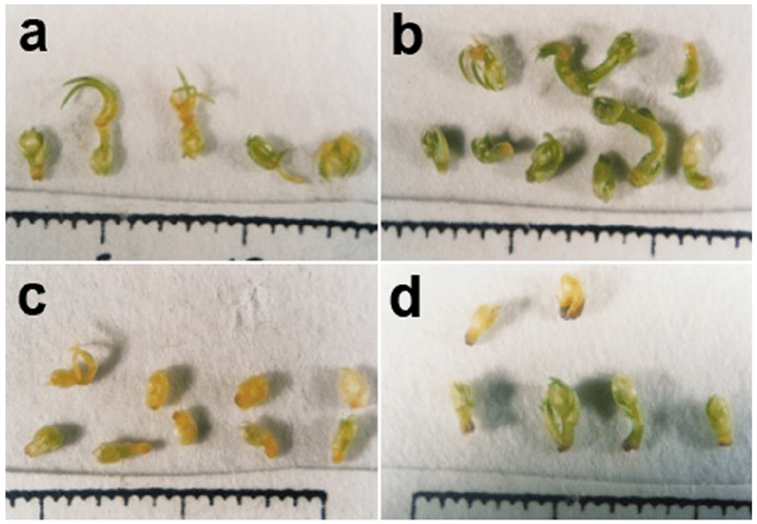
Figure 1. Plant tissues obtained from Asparagus officinalis plant cultures sprouted in various environments7. (a) Growth in Muchison CM2 meteorite (b) Growth in DaG 476 meteorite (c) Growth in water (d) Growth in Hawaii lava Mars simulation.
In addition to that, observation of algae, liken, stromatolite, fossilized algae, and samples like metazoans on Mars or observation of samples like terrestrial fungus in Mars and Venus, supports the hypothesis that the origin of life started in space and spread to Earth20,21. Nutritional values of exoplanet materials were shown on a synthetic terrestrial analog tholin, towards the end of 20th century22. In 1997, it was observed that Murchison CM2 meteorite has fertility parameters of terrestrial soil23. Additionally, it has been reported that Murchison materials can support the life of anaerobic and aerobic thermophile species24. Studies made with extremophiles, which can survive in extreme environments, stand out as another species that would expand our understanding of life on exoplanets25. It is also possible to examine the samples collected in extraterrestrial potential ecosystem studies, especially in space missions26,27. In a study made by ExoMars rover in 1996, formulated ESA’s (Europe Space Agency) searches and guidelines for future life in the solar system28,29. It has been thought that life existed in the first half million years after the formation of Mars. The environment of microorganisms was similar to the early Earth. These reasons make Mars the most appropriate planet to search for signs of life in the solar system30,31. MARSBOx (Microbes in Atmosphere for Radiation, Survival, and Biological Outcome Experiment) is an experiment that started in 2019 to search for terrestrial life on other planets by sending fungi spores of Aspergillus niger, Salinisphaera shabanensis, Staphylococcus capitis subsp. kapitisand Buttiauxella sp. in NASA science balloon to the Earth’s middle stratosphere where conditions are like far planets. MARSBOx model has atmosphere and pressure conditions of Mars, is designed by modifying Europe Space Agents’ (ESA) transport and exposure box (Trex-Box), which was used in EXPOSE-E, EXPOSE-R, and MASE (Mars Analogues for Space Expeditions) for biological exposure research. The results of experiments on specimens (Figure 2)32 that are prepared by changing ¼th of each layer of Trex-Box to make it more compatible with the Martian environment, indicate that bacteria can adapt to the Mars environment in different ways, and fungal spores can be resistant to possible migration to other planets32. A study made with microorganisms that can be beneficial to ecological restoration and space research showed that Escherichia coli in a stimulated Lower Earth Orbit (LEO) environment has survival abilities against DNA damage and we are closer to engineering new bacteria species that can adapt to space environment33. Studies to observe the survivability of microorganisms in outer space and adaptation to the space environment continue with the guided evolution method34-36.
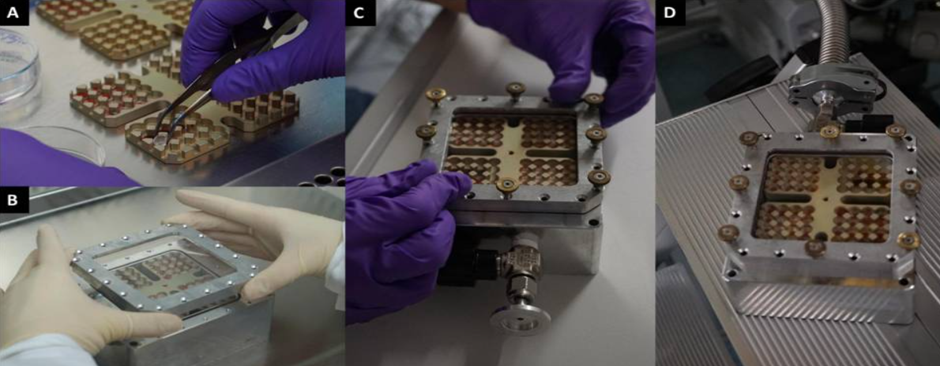
Figure 2. Steps for Trex-Box specimen preparation32. Trex-Box is a gas-tight box that allows alteration of the inner atmosphere by being perforated. (A) Quartz discs that contain microbial samples in Trex-Box (B) Sealing the Trex-Box with suprasil glass that allows passage of UV-VIS (C) Using screws to tighten and secure the container (D) Replacing Earth’s atmosphere in the Trex-box with Mars’s gas mixture.
Sustainability and Worldization (Terraforming)
Environmental sustainability is defined as the degree of sustainability of a process or initiative while avoiding the long-term depletion of natural resources37,38. The rapid change in ecosystems and Earth conditions due to human activities has revealed the importance of ecological sustainability research39. A sustainable development approach to managing potential ecological crises is created to support sustainability on Earth and in space exploration40,41. Bioregenerative Life Support Systems (BLSS) are designed for the sustainability of life in the space environment in artificial closed ecosystems that contain humans, plants, animals, and microorganisms42. The design of the BLSS is based on a combination of ecological principles and technology and currently has a key role in space exploration43,44. Worldization (Terraforming) is a term that is used to change a hostile planet environment to an Earth-like environment45. Planetary ecosynthesis is defined as the alteration of another planet’s environment on the purpose of increasing the survival rate of local biology46. Mars is currently the most suitable candidate for terraforming47,48.
Astroecology and Protection of Species
Astroecology aims to facilitate the monitoring of ecosystem dynamics for the sustainability of species. Conservation science benefits from astroecology to identify endangered species, eliminate problems in their conservation, and monitor their populations on land and sea49,50. Astroecology is a branch of science that studies ecosystems and interactions with space conditions as an interdisciplinary approach. Even though there are various indicators that the origin of life on Earth came from other planets, definitive evidence on this subject requires combined work of astroecology and engineering disciplines.
References
- Buettel, J. C., Brook, B. W., Cole, A., Dickey, J., & Flies, E. J. (2018). Astro-ecology? Shifting the interdisciplinary collaboration paradigm. Ecology and Evolution, 8(19), 9586–9589. https://doi.org/10.1002/ECE3.4455
- Kleiven, E. F., Henden, J. A., Ims, R. A., & Yoccoz, N. G. (2018). Seasonal difference in temporal transferability of an ecological model: near-term predictions of lemming outbreak abundances. Scientific Reports 2018 8:1, 8(1), 1–6. https://doi.org/10.1038/s41598-018-33443-6
- Lauesen, L. M. (2013). Ecology. Encyclopedia of Corporate Social Responsibility, 885–892. https://doi.org/10.1007/978-3-642-28036-8_381
- Yu, G. R., Wang, Q. F., Yang, M., & Chen, Z. (2021). [Discussion on the scientific concept of ecology and its evolution and the contemporary ecologi-cal discipline system]. Ying Yong Sheng Tai Xue Bao = The Journal of Applied Ecology, 32(1), 1–15. https://doi.org/10.13287/J.1001-9332.202101.040
- Watts, E., Hoßfeld, U., & Levit, G. S. (2019). Ecology and Evolution: Haeckel’s Darwinian Paradigm. Trends in Ecology and Evolution, 34(8), 681–683. https://doi.org/10.1016/j.tree.2019.04.003
- Mautner, M. (2005). Life in the Cosmological Future: Resources, Biomass and Populations. J. British Interplanetary Soc., 58, 167-180.
- Mautner, M. N. (2014). Astroecology, cosmo-ecology, and the future of life. Acta Societatis Botanicorum Poloniae, 83(4), 449–464. https://doi.org/10.5586/asbp.2014.036
- Nielsen, S. N., Müller, F., Marques, J. C., Bastianoni, S., & Jørgensen, S. E. (2020). Thermodynamics in Ecology—An Introductory Review. Entropy 2020, Vol. 22, Page 820, 22(8), 820. https://doi.org/10.3390/E22080820
- Cohen, I. R., Marron, A., Rosenberg, E., & Koonin, E. V. (2020). The evolution of universal adaptations of life is driven by universal properties of matter: energy, entropy, and interaction.
- F1000research, 9, 626-626. https://doi.org/10.12688/F1000RESEARCH.24447.3
- Lu, H., Fu, F., Li, H., Campbell, D. E., & Ren, H. (2015). Eco-exergy and emergy based self-organization of three forest plantations in lower subtropical China. Scientific Reports, 5. https://doi.org/10.1038/SREP15047
- Yadav, M., Kumar, R., & Krishnamurthy, R. (2020). Chemistry of Abiotic Nucleotide Synthesis. Chemical Reviews, 120(11), 4766–4805. https://doi.org/10.1021/ACS.CHEMREV.9B00546
- Steele, E. J. (2020). Introduction—Panspermia, 2020. Advances in Genetics, 106, 1. https://doi.org/10.1016/BS.ADGEN.2020.04.001
- Wickramasinghe, N. C., Wickramasinghe, D. T., & Steele, E. J. (2018). Comets, Enceladus and panspermia. Astrophysics and Space Science 2018 363:12, 363(12), 1–7. https://doi.org/10.1007/S10509-018-3465-0
- Sadlok, G. (2020). On A Hypothetical Mechanism of Interstellar Life Transfer Trough Nomadic Objects. Origins of Life and Evolution of Biospheres, 50(1–2), 87–96. https://doi.org/10.1007/s11084-020-09591-z
- Foreword. (2021). Ultraviolet Astronomy and the Quest for the Origin of Life, xi–xii. https://doi.org/10.1016/B978-0-12-819170-5.06001-2
- Moissl-Eichinger, C., Cockell, C., & Rettberg, P. (2016). Venturing into new realms? Microorganisms in space. FEMS Microbiology Reviews, 40(5), 722–737. https://doi.org/10.1093/FEMSRE/FUW015
- Lingam, M., & Loeb, A. (2017). Enhanced interplanetary panspermia in the TRAPPIST-1 system. Proceedings of the National Academy of Sciences of the United States of America, 114(26), 6689–6693. https://doi.org/10.1073/pnas.1703517114
- von Hegner, I. (2021). Evolutionary Processes Transpiring in the Stages of Lithopanspermia. Acta Biotheoretica 2021 69:4, 69(4), 783–798. https://doi.org/10.1007/S10441-021-09411-5
- Prasad, B., Richter, P., Vadakedath, N., Haag, F. W. M., Strauch, S. M., Mancinelli, R., Schwarzwälder, A., Etcheparre, E., Gaume, N., & Lebert, M. (2021). How the space environment influences organisms: an astrobiological perspective and review. International Journal of Astrobiology, 20(2), 159–177. https://doi.org/10.1017/S1473550421000057
- Joseph, R. G., Planchon, O., Duxbury, N. S., Latif, K., Kidron, G. J., Consorti, L., Armstrong, R. A., Gibson, C., & Schild, R. (2020). Oceans, Lakes, and Stromatolites on Mars. Advances in Astronomy, 2020. https://doi.org/10.1155/2020/6959532
- Joseph, R. G., Planchon, O., Gibson, C. H., & Schild, R. (2020). Seeding the Solar System with Life: Mars, Venus, Earth, Moon, Protoplanets. Open Astronomy, 29(1), 124–157. https://doi.org/10.1515/ASTRO-2020-0019
- McDonald, G. D., Khare, B. N., Reid Thompson, W., & Sagan, C. (1991). CH4/NH3/H2O spark tholin: Chemical analysis and interaction with Jovian aqueous clouds. Icarus, 94(2), 354–367. https://doi.org/10.1016/0019-1035(91)90234-K
- Mautner, M. N., Conner, A. J., Killham, K., & Deamer, D. W. (1997). Biological Potential of Extraterrestrial Materials. Icarus, 129(1), 245–253. https://doi.org/10.1006/ICAR.1997.5786
- Mautner, M. N. (2002). Planetary Bioresources and Astroecology: 1. Planetary Microcosm Bioassays of Martian and Carbonaceous Chondrite Materials: Nutrients, Electrolyte Solutions, and Algal and Plant Responses. Icarus, 158(1), 72–86. https://doi.org/10.1006/ICAR.2002.6841
- Merino, N., Aronson, H. S., Bojanova, D. P., Feyhl-Buska, J., Wong, M. L., Zhang, S., & Giovannelli, D. (2019). Living at the extremes: Extremophiles and the limits of life in a planetary context. Frontiers in Microbiology, 10(MAR), 780. https://doi.org/10.3389/FMICB.2019.00780
- Chan, Q. H. S., Stroud, R., Martins, Z., & Yabuta, H. (2020). Concerns of Organic Contamination for Sample Return Space Missions. Space Science Reviews, 216(4). https://doi.org/10.1007/S11214-020-00678-7
- Coelho, L. F., Blais, M. A., Matveev, A., Keller-Costa, T., Vincent, W. F., Costa, R., Martins, Z., & Canário, J. (2022). Contamination analysis of Arctic ice samples as planetary field analogs and implications for future life-detection missions to Europa and Enceladus. Scientific Reports 2022 12:1, 12(1), 1–13. https://doi.org/10.1038/s41598-022-16370-5
- Vago, J. L., Westall, F., Coates, A. J., Jaumann, R., Korablev, O., Ciarletti, V., Mitrofanov, I., Josset, J. L., De Sanctis, M. C., Bibring, J. P., Rull, F., Goesmann, F., Steininger, H., Goetz, W., Brinckerhoff, W., Szopa, C., Raulin, F., Edwards, H. G. M., Whyte, L. G., … Carreau, C. (2017). Habitability on Early Mars and the Search for Biosignatures with the ExoMars Rover. Astrobiology, 17(6–7), 471. https://doi.org/10.1089/AST.2016.1533
- Miles, H. C., Gunn, M. D., & Coates, A. J. (2020). Seeing through the “Science Eyes” of the ExoMars Rover. IEEE Computer Graphics and Applications, 40(2), 71–81. https://doi.org/10.1109/MCG.2020.2970796
- Westall, F., Loizeau, D., Foucher, F., Bost, N., Betrand, M., Vago, J., & Kminek, G. (2013). Habitability on Mars from a Microbial Point of View. Https://Home.Liebertpub.Com/Ast, 13(9), 887–897. https://doi.org/10.1089/AST.2013.1000
- Siela, A. C., & Smith, S. A. (2019). Habitability of Mars: How Welcoming Are the Surface and Subsurface to Life on the Red Planet? Geosciences 2019, Vol. 9, Page 361, 9(9), 361. https://doi.org/10.3390/GEOSCIENCES9090361
- Cortesão, M., Siems, K., Koch, S., Beblo-Vranesevic, K., Rabbow, E., Berger, T., Lane, M., James, L., Johnson, P., Waters, S. M., Verma, S. D., Smith, D. J., & Moeller, R. (2021). MARSBOx: Fungal and Bacterial Endurance From a Balloon-Flown Analog Mission in the Stratosphere. Frontiers in Microbiology, 12. https://doi.org/10.3389/FMICB.2021.601713
- Puig, J., Knödlseder, N., Quera, J., Algara, M., & Güell, M. (2021). DNA Damage Protection for Enhanced Bacterial Survival Under Simulated Low Earth Orbit Environmental Conditions in Escherichia coli. Frontiers in Microbiology, 12. https://doi.org/10.3389/FMICB.2021.789668
- Bruckbauer, S. T., Trimarco, J. D., Martin, J., Bushnell, B., Senn, K. A., Schackwitz, W., Lipzen, A., Blow, M., Wood, E. A., Culberson, W. S., Pennacchio, C., & Cox, M. M. (2019). Experimental Evolution of Extreme Resistance to Ionizing Radiation in Escherichia coli after 50 Cycles of Selection. Journal of Bacteriology, 201(8). https://doi.org/10.1128/JB.00784-18
- Bruckbauer, S. T., Martin, J., Minkoff, B. B., Veling, M. T., Lancaster, I., Liu, J., Trimarco, J. D., Bushnell, B., Lipzen, A., Wood, E. A., Sussman, M. R., Pennacchio, C., & Cox, M. M. (2020). Physiology of Highly Radioresistant Escherichia coli After Experimental Evolution for 100 Cycles of Selection. Frontiers in Microbiology, 11. https://doi.org/10.3389/FMICB.2020.582590
- Bruckbauer, S. T., & Cox, M. M. (2021). Experimental evolution of extremophile resistance to ionizing radiation. Trends in Genetics: TIG, 37(9), 830–845. https://doi.org/10.1016/J.TIG.2021.04.011
- Federation, F. W. D. (2018). Sustainability in Dentistry: Adopted by the FDI General Assembly: August 2017, Madrid, Spain. International Dental Journal, 68(1), 10. https://doi.org/10.1111/IDJ.12369
- Oláh, J., Aburumman, N., Popp, J., Khan, M. A., Haddad, H., & Kitukutha, N. (2020). Impact of Industry 4.0 on Environmental Sustainability. Sustainability 2020, Vol. 12, Page 4674, 12(11), 4674. https://doi.org/10.3390/SU12114674
- Lubowiecki-Vikuk, A., Dąbrowska, A., & Machnik, A. (2021). Responsible consumer and lifestyle: Sustainability insights. Sustainable Production and Consumption, 25, 91. https://doi.org/10.1016/J.SPC.2020.08.007
- History, J. A. D. P. P. of. (2007). Sustainable development– historical roots of the concept. Https://Doi.Org/10.1080/15693430600688831, 3(2), 83–96. https://doi.org/10.1080/15693430600688831
- Maiwald, V., Schubert, D., Quantius, D., & Zabel, P. (2021). From space back to Earth: supporting sustainable development with spaceflight technologies. Sustainable Earth 2021 4:1, 4(1), 1–16. https://doi.org/10.1186/S42055-021-00042-9
- Liu, H., Yao, Z., Fu, Y., & Feng, J. (2021). Review of research into bioregenerative life support system(s) which can support humans living in space. Life Sciences in Space Research, 31, 113–120. https://doi.org/10.1016/J.LSSR.2021.09.003
- Irons, M. A., & Irons, L. G. (2021). Terraform Sustainability Assessment Framework for Bioregenerative Life Support Systems. Frontiers in Astronomy and Space Sciences, 8, 214. https://doi.org/10.3389/FSPAS.2021.789563
- Häder, D. (2019). On the Way to Mars—Flagellated Algae in Bioregenerative Life Support Systems Under Microgravity Conditions. Frontiers in Plant Science, 10. https://doi.org/10.3389/FPLS.2019.01621
- Solé, R. V., Montañez, R., Duran-Nebreda, S., Rodriguez-Amor, D., Vidiella, B., & Sardanyés, J. (2018). Population dynamics of synthetic terraformation motifs. Royal Society Open Science, 5(7). https://doi.org/10.1098/RSOS.180121
- McKay, C. P. (2011). Planetary Ecosynthesis. Encyclopedia of Astrobiology, 1268–1269. https://doi.org/10.1007/978-3-642-11274-4_1569
- Vaz, E., & Penfound, E. (2020). Mars Terraforming: A Geographic Information Systems Framework. Life Sciences in Space Research, 24, 50–63. https://doi.org/10.1016/J.LSSR.2019.12.001
- Conde-Pueyo, N., Vidiella, B., Sardanyés, J., Berdugo, M., Maestre, F. T., Lorenzo, V. de, & Solé, R. (2020). Synthetic Biology for Terraformation Lessons from Mars, Earth, and the Microbiome. Life 2020, Vol. 10, Page 14, 10(2), 14. https://doi.org/10.3390/LIFE10020014
- Burke, C., Rashman, M. F., McAree, O., Hambrecht, L., Longmore, S. N., Piel, A. K., & Wich, S. A. (2018). Addressing environmental and atmospheric challenges for capturing high-precision thermal infrared data in the field of astro-ecology. 63. https://doi.org/10.48550/arxiv.1807.03157
- McWhirter, P. R., Veitch-Michaelis, J., Burke, C., Lam, M. C., Longmore, S. N., McWhirter, P. R., Veitch-Michaelis, J., Burke, C., Lam, M. C., & Longmore, S. N. (2019). Saving Endangered Animals with AstroEcology. ASPC, 523, 95. https://ui.adsabs.harvard.edu/abs/2019ASPC..523…95M/abstract